Conformational Dynamics of Proteins

Proteins are polypeptides which consist of typically 50 to several 100 amino acids and fold into protein-specific three-dimensional structures. This native structure is determined by the amino acid sequence of a protein, which is genetically encoded. A protein is not rigid, however. Rather, it can undergo a variety of (fast) vibrations and (slower) structural rearrangements, the latter being called 'conformational transitions'.
This situation is best summarized by a one-dimensional sketch of the complex, high-dimensional energy landscape (see Figure), which determines the correspondingly complex dynamics of a protein, as originally suggested by Hans Frauenfelder. This energy landscape is characterized by a multitude of almost iso-energetic minima, which are separated from each other by energy barriers of various heights. Each of these minima corresponds to one particular protein structure ('conformational substate'); neighboring minima correspond to similar structures. Structural transitions are barrier crossings (arrows), and the transition rate is determined by the height of the barrier.

Since in conventional molecular dynamics simulations only few nanoseconds can be covered, only the smallest barriers are overcome in simulations, and the observed structural changes are small (bottom right arrow and inset). The larger barriers are traversed more rarely (however the transition process itself may well be fast), and thus are not observed in molecular dynamics simulations. We have developed a method called 'conformational flooding' [1,2], which accelerates conformational transitions in molecular dynamics simulations by several orders of magnitude and thereby actually can bring slow conformational transitions into the scope of simulations. This work was done at the Institut für Medizinische Optik, Univerity of Munich.
'Conformational flooding' intimately links concepts of statistical mechanics to MD simulations and is mathematically involved, but the basic idea is simple (see Figure): In a typical nanosecond simulation, the structure is stuck in one of the minima of the high dimensional energy landscape discussed above (top of Figure). Thus, the question of in what direction of the huge number of possibilities in the high-dimensional conformational space the structure is going to cross a barrier (arrow) at, say, a millisecond time scale, cannot be answered in the first place.
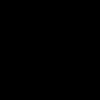
However, from the ensemble generated by the simulation one can construct a localized artificial 'flooding potential' of certain, but variable strength (mid). This potential is included within subsequent 'flooding simulations' and rises the minimum of the initial conformation. Thereby, the barrier hight is reduced, and, following transition state theory, the transitions are accelerated. It is important to note that this behaviour is achieved solely by modifying the energy landscape within the minimum - where we know the dynamics already and thus are no longer interested in it; as can be seen from the Figure, the barriers and all the other minima - which we are interested in - are not modified at all.
The bottom-line is that 'conformational flooding' is expected to induce unbiased transitions, i.e., those which would be observed without the flooding potential, too, at a much longer time scale.