Differential Peptide Dynamics is Linked to MHC Polymorphism
Rainer Böckmann and Helmut Grubmüller
Collaborating groups: Alexiev and Ziegler (FU Berlin)
Supported by: Max Planck Gesellschaft, European Union
The presentation of peptides by major histocompatibility complex (MHC) molecules is the primary event of immune responses. Peptidic fragments of self or non-self proteins are loaded on MHC class I molecules in the endoplasmatic reticulum, followed by transport to the cell surface. Recognition of peptide-loaded MHC molecules by T-cell receptors (TCR) triggers an immune response. Despite their inherent crossreactivity, TCR must be able to discriminate between foreign antigens and the huge number of self-peptides to avoid autoreactivity. How the recognition processes are accomplished in molecular terms is just beginning to be understood. We addressed this issue by a combined time-resolved fluorescence depolarization and molecular dynamics simulations of two human MHC class I subtypes (Figure). Despite virtually identical structures, we found pronounced peptide flexibility only for the non-disease associated subtype, suggesting an entropic control of peptide recognition. This finding was also supported by calorimetric measurements.
The two studied human leucocyte antigen (HLA) class I subtypes are differentially associated with the autoimmune disease ankylosing spondylitis. HLA-B*2705 and B*2709 differ only in one amino acid at position 116 at the floor of the peptide binding groove. High resolution X-ray structures of the soluble ectodomains of both subtypes in complex with the model peptide m9 (GRFAAAIAK) have previously been determined and are virtually indistinguishable. To investigate possible differences in the dynamics and motional freedom of the m9 peptide, as well as the thermodynamic stability of the protein-MHC-complex (pMHC), we applied time-resolved fluorescence depolarization and molecular dynamics simulations.
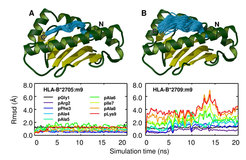
As can be seen in the Figure, the peptide displays a dramatically enhanced flexibility only when bound to B*2709 (right), whereas the conformational bundle is narrow in B*2705 (left). As can be seen from the root mean squared fluctuations (bottom), also obtained from the simulations, the subtype-dependent conformational heterogeneity increases towards the C-terminus of the peptide. The primary anchor residue pArg2, which is common among HLA-B27 subtypes, is tightly bound in both subtypes (black line). Notably, the increased flexibility of the peptide imposes an increased flexibility also on the C-terminal part of the alpha-1 helix, which forms half of the binding pocket.
The different flexibilities of the peptide and its binding grooves, as seen in the simulations and time-resolved experiments, explain measured entropy changes for thermally induced peptide dissociation.
Taken together, our study shows that similar pMHC structures may exhibit drastic HLA-subtype-dependent dynamical differences, even when the subtypes are distinguished only by a deeply buried polymorphism. Moreover, binding of TCR or other ligands on an effector cell to a pMHC should at least partly be controlled by pMHC flexibility, thus influencing effector cell responses. Accordingly, by entropically contributing to the binding and unbinding kinetics, the degree of pMHC flexibility can in particular control optimal T cell recognition and activation via the half-life of the pMHC-TCR interaction. We propose this mechanism as the atomistic basis for the entropic control of pMHC recognition with MHC polymorphism linked entropic peptide presentation thresholds.