Analysis of cytoskeletal organization and function in oocyte meiosis
Many essential steps of meiosis are driven by cytoskeletal structures. These cytoskeletal structures often differ from their seemingly more reliable mitotic counterparts. Thus, differences in cytoskeletal organization might contribute to meiotic errors. For instance, chromosome segregation is driven by a specialized meiotic microtubule spindle that – in contrast to mitotic spindles - lacks centrosomes at its poles (Manandhar et al., 2005); and asymmetric positioning of the spindle in mouse oocytes is actin- instead of microtubule-dependent as in most mitotic cells (Longo and Chen, 1985). A growing body of evidence suggests that actin and myosins also have important functions for chromosome segregation and spindle assembly during meiosis in various organisms (Sandquist et al., 2011). For instance, actin is required for chromosome congression in starfish oocytes (Lenart et al., 2005) and a myosin is essential for spindle assembly in Xenopus oocytes (Sandquist et al., 2011). Whether actin and myosins have similar functions in mammalian oocytes remains to be investigated.
Over the past few years, we have made significant progress in analyzing essential functions of actin in mouse oocytes. In addition, we have studied how the microtubule spindle is organized in mammalian oocytes and at the oocyte-to-embryo transition. This includes the first studies of spindle assembly in live human oocytes (see Study meiosis and causes of aneuploidy in human oocytes).
New functions of actin in mammalian oocytes
Actin promotes accurate chromosome alignment and segregation in mouse oocytes
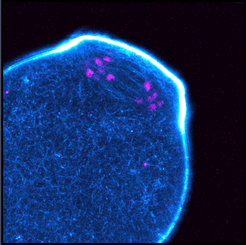
We found that prominent actin filaments permeate the acentrosomal microtubule spindle in oocytes of several other mammalian species, including humans (Fig. 1). We demonstrated that actin promotes the accurate alignment and segregation of chromosomes. Oocytes in which the actin spindle in disrupted display a significantly increased number of misaligned chromosomes as well as lagging chromosomes during meiosis I and II. We show that actin safeguards chromosome alignment and segregation by promoting the formation of kinetochore fibres, which mediate the alignment and segregation of chromosomes. These findings establish an essential role for actin in meiotic chromosome segregation and suggest a new principle of how kinetochore fibres are established (Mogessie and Schuh, Science 2017).
Functions of actin in asymmetric spindle positioning and vesicle transport
Much of our work has focused on understanding how actin helps to position the spindle asymmetrically before the extrusion of the first polar body (Fig. 2). This is an important question because asymmetric spindle positioning is a prerequisite for the extremely asymmetric division of the oocyte, which ensures that the egg retains sufficient storage material for embryo development (Fig. 3).
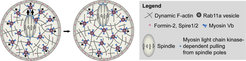
Figure 2. Mechanism of actin-dependent spindle and vesicle transport. Past work in our group revealed that asymmetric spindle positioning is driven by a cytoplasmic actin network that is nucleated by cooperation between the actin nucleation factors Formin-2 and Spire1/2. Spindle movement along this network is facilitated by myosin-dependent pulling from the spindle poles and a outward-directed myosin Vb-dependent movement of Rab11a-positive vesicles, which keep the network dynamic and help to modulate its density by localizing and transporting the actin nucleation factors.
Spire-type actin nucleators cooperate with Formin-2 to drive asymmetric oocyte division
Our previous work established that asymmetric spindle positioning in mouse oocytes requires a cytoplasmic actin network that is assembled by the actin nucleation factor Formin-2. The spindle pulls on this network while it moves to the oocyte’s surface in a myosin-dependent manner (Schuh and Ellenberg, 2008). We found that Spire-type actin nucleation factors cooperate with Formin-2 to drive the asymmetric division of mouse oocytes. In particular, Spire proteins and Formin-2 rely on each other to assemble the cytoplasmic actin network that mediates asymmetric spindle positioning and are essential for ingression of the cytokinetic furrow. Depletion of Spire proteins results in diploid eggs that cannot give rise to euploid embryos upon fertilization (Pfender et al., 2011).
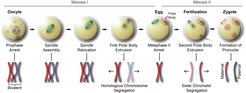
Figure 3. Meiosis and fertilization in mammalian oocytes. In the upper row, the different stages of meiosis in mammalian oocytes and the corresponding large scale chromosome (red) and microtubule (green) organization are shown. In the lower row, the detailed configuration of a pair of homologous metacentric chromosomes is shown.
Spire and Formin-2 synergize and antagonize in regulating actin assembly in meiosis by a ping-pong mechanism
We further investigated the cooperation between Spire-type actin nucleation factors and Formin-2 in vitro in collaboration with Marie-France Carlier’s group. This work revealed that Spire and Formin-2 assemble F-actin by a ping-pong mechanism, in which the actin filament is repetitively passed between the two proteins during assembly (Montaville et al., 2014).
An actin-dependent mechanism for long-range vesicle transport
We have also investigated other functions of the actin network in the oocyte. Surprisingly, we found that it is required to transport vesicles over long distances. In particular, we found that Rab11a-positive vesicles move directionally along the network in a myosin-Vb-dependent manner to converge and to reach the cell surface. Microtubules were dispensable for vesicle movement. This was unexpected because long-range transport of vesicles was generally thought to be microtubule-dependent (Schuh, 2011).
Vesicles modulate an actin network for asymmetric spindle positioning
Moreover, we found that the vesicles not only move along the actin network, but that oocytes use the vesicles as adaptable, motorized network nodes to regulate the dynamics and density of the network. In particular, Rab11a-positive vesicles drive the network dynamics in a myosin-Vb-dependent manner, and help to modulate the network density by sequestering and clustering the network's actin nucleators. Our work also revealed a simple way by which networks of different densities can be generated, namely by adjusting the number and volume of vesicles in the cell. This vesicle-based mechanism of actin network modulation is essential for asymmetric positioning of the meiotic spindle in mouse oocytes (Holubcova et al., 2013).
Transport of cortical granules along a cytoplasmic actin network prevents polyspermy
An egg must be fertilised by a single sperm only. To prevent polyspermy, the zona pellucida, a structure that surrounds mammalian eggs, becomes impermeable upon fertilisation, preventing the entry of further sperm. The structural changes in the zona upon fertilization are driven by the exocytosis of cortical granules. These translocate from the oocyte’s centre to the plasma membrane during meiosis. However, very little is known about the mechanism of cortical granule translocation. We investigated cortical granule transport and dynamics for the first time in live mammalian oocytes by using Rab27a as a marker. We found that two separate mechanisms drive their transport: myosin Va-dependent movement along actin filaments, and an unexpected vesicle hitchhiking mechanism by which cortical granules bind to Rab11a vesicles powered by myosin Vb. Inhibiting cortical granule translocation severely impaired the block to sperm entry, suggesting that translocation defects could contribute to miscarriages that are caused by polyspermy (Cheeseman et al., Nat. Comm. 2016).
Meiotic spindle organization
Our work also focussed on how the acentrosomal meiotic spindle is organized in mammalian oocytes. This topic is of particular relevance because our work in human oocytes suggests that multipolar spindle intermediates and spindle instability, which are a frequent phenomenon during spindle assembly in human oocytes, correlate with chromosome segregation errors (Holubcova et al., 2015; see Meiosis and causes of aneuploidy in human oocytes).
MTOC fragmentation safeguards spindle bipolarity and chromosome congression in mouse oocytes
Spindle assembly in mouse oocytes is driven by self-organization of numerous acentriolar microtubule organizing centers (MTOCs) (Schuh and Ellenberg, 2007). Recent work from our lab has revealed that mouse oocytes need to fragment the MTOCs into a high number of small MTOCs to be able to then regroup and merge them into two equal spindle poles. We found that MTOCs are fragmented in a 3-step process. First, Plk1 triggers a loosening of MTOC structure that involves the release of the centrosomal linker protein C-Nap1. Second, BicD2-anchored Dynein stretches the MTOCs into fragmented ribbons on the surface of the nuclear envelope. Third, KIF-11 further fragments the MTOCs following nuclear envelope breakdown so that they can be evenly distributed towards the two spindle poles. Blocking MTOC fragmentation leads to defects in spindle assembly, which delay chromosome individualization and congression (Clift and Schuh, 2015).
The transition from meiotic to mitotic spindle assembly proceeds gradually during early mammalian development
In collaboration with Takashi Hiiragi’s lab at EMBL Heidelberg, we have studied the mechanism of spindle assembly in early mouse embryos. This work revealed a surprising gradual transition from meiosis to mitosis over the first eight divisions of the mouse embryo: spindle assembly in the mouse zygote is achieved by a similar mechanism as in mouse oocytes, and only gradually, the spindle adapts the properties of a centrosomal spindle found in typical mitotic cells (Courtois et al., 2012).
References
Cheeseman, L.P., Boulanger, J., Bond, L.M., and Schuh, M. (2016). Two pathways regulate cortical granule translocation to prevent polyspermy in mouse oocytes. Nat. Commun. 7, 13726.
Clift, D., and Schuh, M. (2015). A 3-step MTOC fragmentation mechanism facilitates bipolar spindle assembly in mouse oocytes. Nat Comm 6:7217.
Courtois, A., Schuh, M., Ellenberg, J., and Hiiragi, T. (2012). The transition from meiotic to mitotic spindle assembly is gradual during early mammalian development. J Cell Biol 198, 357-370.
Holubcová, Z., Blayney, M., Elder, K., and Schuh, M. (2015). Error-prone chromosome-mediated spindle assembly favors chromosome segregation defects in human oocytes. Science 348, 1143-1147.
Holubcova, Z., Howard, G., and Schuh, M. (2013). Vesicles modulate an actin network for asymmetric spindle positioning. Nat Cell Biol 15, 937-947.
Lenart, P., Bacher, C.P., Daigle, N., Hand, A.R., Eils, R., Terasaki, M., and Ellenberg, J. (2005). A contractile nuclear actin network drives chromosome congression in oocytes. Nature 436, 812-818.
Longo, F.J., and Chen, D.Y. (1985). Development of cortical polarity in mouse eggs: involvement of the meiotic apparatus. Dev Biol 107, 382-394.
Manandhar, G., Schatten, H., and Sutovsky, P. (2005). Centrosome reduction during gametogenesis and its significance. Biol Reprod 72, 2-13.
Mogessie, B., and Schuh, M. (2017). Actin protects mammalian eggs against chromosome segregation errors. Science 357, eaal1647.
Montaville, P., Jegou, A., Pernier, J., Compper, C., Guichard, B., Mogessie, B., Schuh, M., Romet-Lemonne, G., and Carlier, M.F. (2014). Spire and Formin 2 synergize and antagonize in regulating actin assembly in meiosis by a ping-pong mechanism. PLoS Biol 12, e1001795.
Pfender, S., Kuznetsov, V., Pleiser, S., Kerkhoff, E., and Schuh, M. (2011). Spire-type actin nucleators cooperate with Formin-2 to drive asymmetric oocyte division. Current biology : CB 21, 955-960.
Sandquist, J.C., Kita, A.M., and Bement, W.M. (2011). And the dead shall rise: actin and myosin return to the spindle. Dev Cell 21, 410-419.
Schuh, M. (2011). An actin-dependent mechanism for long-range vesicle transport. Nature cell biology 13, 1431-1436.
Schuh, M., and Ellenberg, J. (2007). Self-organization of MTOCs replaces centrosome function during acentrosomal spindle assembly in live mouse oocytes. Cell 130, 484-498.
Schuh, M., and Ellenberg, J. (2008). A new model for asymmetric spindle positioning in mouse oocytes. Curr Biol 18, 1986-1992.
