State-to-state molecular beam surface scattering
The group’s direction of work lies in the field of experimental physical chemistry. Being part of the “Dynamics at Surface” group headed by Alec Wodtke, the research direction focuses on the dynamics of molecules at the gas-surface interface. As experimental approach we employ molecular beam surface scattering experiments: we scatter molecular beams from well-defined surfaces under ultra-high vacuum conditions and prepare and detect scattered molecules using laser spectroscopy
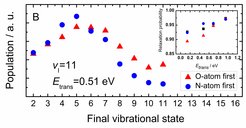
Figure 1: Final vibrational state distribution for scattering NO(v=11) from a Au(111) surface with different molecular orientations. The inset shows the relaxation probability for NO(v=11) at different incident translational energies.
In the past, we intensively studied the non-reactive interaction of diatomic molecules at several surfaces.1-2 Driven by the idea to understand the influence of electronically excited surface electrons on the molecule-surface interaction, the dynamics of molecular degrees of freedom (translation, rotation, vibration) in surface collisions have been studied. As the energy transfer between the degrees of freedom is critical for chemical reactions at surfaces, these fundamental studies are important for a detailed understanding of heterogeneous catalysis. In particular, molecular adsorption at surfaces and the stretching of molecular bonds (molecular vibrational dynamics) is often the first step of chemical reactions at surfaces. Accordingly, we have placed emphasis on these topics in recent years.
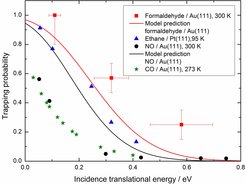
Figure 2: Sticking/Trapping probabilities of different systems as function of the incidence translational energy for systems with similar masses. The sticking probability for polyatomic molecules is significantly enhanced when comparing to diatomic molecules.
We developed a new method for molecular orientation and studied steric effects when scattering NO molecules from metal surfaces.1 The results of this work have triggered several theoretical studies describing surface dynamics beyond the Born Oppenheimer Approximation (BOA). Figure 1 displays the final vibrational state distribution of NO(v=11) molecules after surface scattering from a Au(111) surface for two different orientations. Due to increased non-adiabatic interactions the relaxation probability is higher when scattering the molecules with the N atom first. In addition, the relaxation probability increases at elevated incidence translational energies as shown in the inset of Figure 1. In addition, we designed a new machine combining a Stark decelerator with a UHV surface science chamber. The Stark decelerator allows for complete control of the incidence molecular beam employing the linear Stark effect of neutral polar molecules. With this machine we investigated processes of electronically excited CO molecules at surfaces.
Recently, the focus of our group has been extended towards an understanding of surface dynamics of polyatomic molecules.3-4 For this, we developed a new spectroscopic approach for detecting formaldehyde in the gas phase employing resonance enhanced multiphoton ionization (REMPI). Employing this technique we studied adsorption dynamics of formaldehyde resolving the influence of translational, rotational and vibrational degrees of freedom.
The sticking probability of formaldehyde is significantly enhanced when comparing to diatomic molecules. Figure 2 plots sticking probabilities of different systems as a function of the incidence translational energy of colliding molecules. All systems have similar masses. It is obvious that polyatomic molecules stick at higher incidence energies than diatomic molecules.
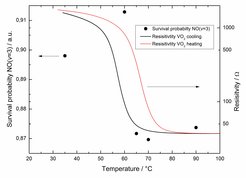
Figure 3: Vibrational state survival probability of NO(v=2) on VO2 as function of the surface temperature. Also shown is the resistivity of VO2 exhibiting hysteresis on a logarithmic scale. At elevated temperatures, VO2 becomes more conducting, and the relaxation of scattering NO(v=3) increases.
In our studies we show that this effect is caused by the increased number of degrees of freedom in polyatomic molecules. In particular, we observe efficient translational to rotational energy transfer which causes non-thermal rotational rainbow excitation in formaldehyde.4 The transfer to rotational degrees of freedom slows the incident molecules down and leads to enhanced trapping at the surface.
Also recently, we performed molecular beam surface scattering at oxide surfaces. Here, we prepared thin films of VO2 using chemical vapor deposition (CVD) methods in collaboration with Prof. Rahinov at the Open University Tel Aviv. VO2 exhibits a Mott transition at 68°C. Below this temperature, VO2 is electrically insulating, whereas it becomes conducting above 68°C. We used such prepared surfaces to study the vibrational energy transfer of NO(v=3) in surface collisions with VO2. Figure 3 shows the relaxation probability of NO(v=3) as well as the resistivity of the VO2 sample as function of the surface temperature. At 68°C, the conductivity of VO2 increases drastically and leads to an enhanced vibrational relaxation probability.
In an additional project, we employ a velocity map imaging detector to record photoelectrons of chiral oxidized hydrocarbons.5 We ionize chiral molecules in a molecular beam by REMPI and image the photoelectron angular distributions (PAD) on a phosphor screen. Due to scattering of the emitted electrons with the chiral molecular frame, the PADs will have an asymmetric shape with respect to the laser propagation axis when ionizing chiral molecules with circular polarized light (CPL). The PADs depend significantly on the chirality of the molecule, meaning that two enantiomeric forms of one molecule produce different PADs. The so called photoelectron circular dichroism (PECD) has been studied in detail in the gas phase employing single photon ionization and multi-photon ionization. In particular, recent experiments employing multi-photon ionization after fs-laser pulse ionization have shown that these experiments can be performed also without large laser facilities, which are needed for single photon ionization.
We have recently constructed a new apparatus with which we can employ this technique to molecular beam surface scattering experiments. Figure 4 displays the experimental setup. With this machine, we are able to detect the chirality of reactants and reaction products after well-controlled molecular beam triggered surface reaction experiments. By changing the surface conditions, we plan to steer the chirality of reaction products.
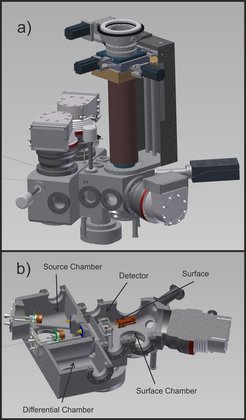
Figure 4: Molecular beam surface scattering apparatus for studies on surface dynamics of aromatic molecules. Panel a) shows the complete apparatus, which is cut out of one metal piece to keep it as compact as possible. Panel b) displays a section view showing the three differentially pumped chambers.
Summarizing, the expertise of my group lies in the field of surface dynamics. We employ molecular beam surface scattering experiments to understand fundamental surface process with relevance to heterogeneous catalysis. In recent years the focus of my group has shifted from simple model systems (diatomic molecules at well-defined surfaces) towards systems of more complexity. In particular, studies on the interaction of polyatomic molecules at surfaces have become increasingly more important in my group.
1. Bartels, N.; Krüger, B. C.; Auerbach, D. J.; Wodtke, A. M.; Schäfer, T., Controlling an Electron-Transfer Reaction at a Metal Surface by Manipulating Reactant Motion and Orientation. Angewandte Chemie International Edition 2014, 53 (50), 13690-13694.
2. Grätz, F.; Engelhart, D. P.; Wagner, R. J. V.; Meijer, G.; Wodtke, A. M.; Schäfer, T., CO (a3Π) quenching at a metal surface: Evidence of an electron transfer mediated mechanism. The Journal of Chemical Physics 2014, 141 (4), 044712.
3. Krüger, B. C.; Park, G. B.; Meyer, S.; Wagner, R. J. V.; Wodtke, A. M.; Schäfer, T., Trapping-desorption and direct-scattering of formaldehyde at Au(111). Physical Chemistry Chemical Physics 2017, 19 (30), 19896-19903.
4. Park, G. B.; Kruger, B. C.; Meyer, S.; Kandratsenka, A.; Wodtke, A. M.; Schafer, T., An axis-specific rotational rainbow in the direct scatter of formaldehyde from Au(111) and its influence on trapping probability. Physical Chemistry Chemical Physics 2017, 19 (30), 19904-19915.
5. Kastner, A.; Ring, T.; Krüger, B. C.; Park, G. B.; Schäfer, T.; Senftleben, A.; Baumert, T., Intermediate state dependence of the photoelectron circular dichroism of fenchone observed via femtosecond resonance-enhanced multi-photon ionization. The Journal of Chemical Physics 2017, 147 (1), 013926.