Fatty acid factory filmed at work
High-resolution images provide new insights into cellular fatty acid production
Fatty acids are essential in all living organisms: to store energy, form membranes, and multiply. They are produced by a complex cellular machinery – the fatty acid synthase (FAS). A team of the Max Planck Institute (MPI) for Multidisciplinary Sciences, led by Ashwin Chari and Holger Stark, have now visualized the FAS structure in unprecedented detail. This allows the direct observation of enzymatic reactions and the reconstruction of structural transitions within a fatty acid synthesis cycle. The findings enable new venues to combat infectious agents and promise advancements in biotechnology.
Humans generally ingest most of their required fatty acids through their diet. Still, fatty acid biosynthesis is a vital metabolic pathway. For yeasts and bacteria, it is even indispensable. Large, multimeric complexes of different enzymes catalyze fatty acid biosynthesis in yeasts and higher organisms, whereas the bacterial counterparts are represented by individual proteins. Although the architecture of the fatty acid biosynthetic machinery varies substantially in different organisms, the catalyzed reactions and individual enzymatic modules resemble each other.
Detailed insight into the structure and chemistry of the fatty acid factory
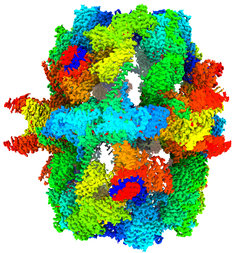
The Max Planck teams led by Holger Stark, head of the Department of Structural Dynamics, and Ashwin Chari, head of the Structural Biochemistry and Mechanisms research group, have now resolved the three-dimensional structure of yeast FAS, for the first, time at an unprecedented resolution: 1.9 angstroms, 19 million times smaller than a millimeter. “In structural biology, surpassing the two-angstrom barrier is essential to understand cellular chemistry,” the Max Planck Director explains. “We reveal the innermost parts of FAS, and can observe both enzymatic reactions and chemical details of how proteins interact with small molecules.”
The combination of biochemistry and high-resolution cryo-electron microscopy was instrumental to the Göttingen scientists’ success. For their experiments, they used the world’s highest-resolution electron microscope which has the ability to resolve individual atoms in a protein.
Visualizing FAS at high precision alone, however, is not sufficient to understand its function. Similar to its human counterpart, fungal FAS synthesizes fatty acids in seven individual reaction steps by using defined chemical precursors in a cyclic, repetitive manner. Every individual chemical step is performed by a separate enzymatic module within FAS. The growing fatty acid chain must therefore be transported from one enzymatic module to the other in an efficient and ordered sequence. A molecular shuttle – the so-called acyl carrier protein (ACP) – carries out this important task and orchestrates the choreography of chemical reactions required for fatty acid biosynthesis.
Observing the molecular shuttle in action

Stark and Chari's teams were also able to film FAS in action and reconstruct a complete fatty acid biosynthesis cycle. For this, the researchers used a combination of methods to follow the ACP on its way through the FAS maze. Initially, they initiated fatty acid biosynthesis in a test-tube, and arrested its activity by rapidly freezing the FAS molecules after different periods of time. This allowed to arrest FAS in distinct states of fatty acid biosynthesis. The cryo-electron microscope then imaged snapshots in the FAS cycle. “Finding the precise combination and amounts of substrates to arrest FAS at critical points in the production cycle was a major technical challenge,” says research group leader Chari. “We can only reconstruct the entire fatty acid biosynthesis cycle if all relevant transitions are visualized and accurately described by models.”
The next step was the computer-aided elucidation of the three-dimensional FAS structures. Kashish Singh, first author of the paper now published in the journal Cell, explains the complex procedure: “We developed image processing procedures that break down FAS into individual functional compartments. We then sorted the structures in a manner that the sequence of images represents a fatty acid biosynthesis cycle. With the help of these snapshots, we were finally able to track how the small ACP molecule interacts with certain sites of FAS and other molecules during fatty acid production.”
Potential for medicine and biotechnology
Meina Neumann-Schaal, Head of Department at the Leibniz Institute German Collection of Microorganisms and Cell Cultures GmbH, reports that this molecule is also medically relevant: “The ACP of yeast FAS contains a structural region that the human counterpart lacks.” This makes the molecule a promising starting point for inhibiting pathogenic organisms that also utilize the yeast-like FAS. These include pathogenic yeast such as Candida albicans which infects mucous membranes, as well as mycobacteria, the infectious agent underlying tuberculosis. As multi-resistant tuberculosis still poses a challenge to successful treatment, there is an urgent need for new inhibitors.
Another finding of the research could potentially be utilized for biotechnological advancements. The teams of Chari and Stark have provided proof-of-concept that additional enzymatic modules can be incorporated into FAS to change its activity. “With normal activity, FAS delivers a mix of short- and long-chain fatty acids. In future, a customized FAS could be used to produce fatty acids of desired chain lengths,” says Chari. These are needed in the chemical industry to produce, among other things, cosmetics, soaps, and flavorings. Notably, these are also building blocks for pharmaceuticals and biofuels. The research teams from Göttingen also see an opportunity to produce fatty acids sustainably by using specifically modified FAS biosynthetic factories, instead of extracting them from crude oil or palm oil as is presently the case. (cr)