Oncophysiology
Electrical signaling is one of the most evident properties of complex living organisms, to the point that we often identify the presence of life with detectable electrical activity, like in the electrocardiogram or the electroencephalogram. Such electrical activity is based by a combination between the differences in the composition of the inside and the outside of the cells, and the presence at the plasma membrane of ion channels. These are proteins capable to respond, within less than a millisecond, to changes in the electrical potential across the plasma membrane. This results in the opening of a pore that allows flow of ions at a rate of over one million per second. Ion channels provide excitable cells with the ability of rapid and reliable signaling, but this is not the only function of ion channels.
In the course of our studies, we identified a particular potassium ion channel whose expression is extensive in the brain but very restricted in other tissues. However, channel expression is abundant in in tumors, and up to 70% human cancers show the channel. In other words, the protein is present in the surface of tumor cells, but not on the neighboring normal cells. We therefore set up to elucidate why tumor cells “switch on” expression, what advantages it gives to the survival of the tumor, and also whether it would be feasible to attack tumors using this differential expression. Our group is also interested in understanding in molecular detail the function of ion channels and the mechanisms implicated. We have structured our studies in three main fields of research:
1. Defining the functions of voltage-gated channels in non-excitable cells, with special attention to mechanisms leading to malignant transformation and tumor progression.
Why is Kv10.1 so often expressed in tumors, and what kind of advantage does it represent for the tumor cell? It is also very important to understand what are the mechanisms responsible for the frequent expression, and the consequences it has for the cells. To tackle this problem, which turns out to be rather complex, we use genetic and cell biological approaches, with emphasis in live cell imaging. We could show that part of the function of the channel has to do with cytoskeletal rearrangements that occur in every cell in many instances, most evidently during cell division. The cytoskeleton is a highly dynamic system in constant reshaping, as it can be seen in the movie (Fig1). A large part of those dynamic changes depend on intracellular calcium, which is influenced by the activity of Kv10.1. We use simultaneous imaging of the cytoskeleton and calcium concentration, particularly in cells undergoing mitosis (Fig2), together with pharmacological and genetic manipulations to dissect the steps of the process and identify the actors implicated. This knowledge is then used to improve our therapeutic approaches and tailor the strategies to the particular conditions of each tumor.
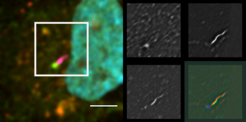
In cells that are not actively dividing, maintenance of the cytoskeleton is also very important. An example of subtle changes triggering profound modifications is the regulation of the growth of the primary cilium, an antenna-like structure that concentrates a large fraction of the signaling mechanism of the cells. We could show that Kv10.1 is implicated in this process, and we can detect the presence of the protein precisely at the primary cilium (Fig3). Another line of research deals with elucidating the mechanisms implicated.
2. Understanding the intimate molecular mechanisms of voltage-gated potassium channel function.
During our biophysical studies, we accidentally observed that cells that we had transfected with this particular ion channel behave in many aspects like malignant cells, a phenomenon that we had not observed with other ion channels. By systematically following this observation, we could demonstrate that Kv10.1 is detected only in the brain in normal conditions, but is abundant in over 70% of tumors. This extraordinary tumor-specificity is the basis of our work in oncology therapeutics. We soon observed that it is possible to reduce tumor growth by inhibiting the activity of the channel, and therefore proposed therapeutic approaches using known drugs (drug repurposing) or antibodies able to inhibit the function of the channel. In fact, treatment with channel blockers improves the survival of brain metastasis patients. The unparalleled tumor specificity of Kv10.1 can also be used for targeting therapeutic agents to the tumor site. Our strategy consists in fusing a protein with therapeutic activity to a single chain antibody or a single domain antibody. In our most advanced construct, TRAIL (TNF-related apoptosis-inducing ligand) is the active moiety fused to the antibody. This construct has proven efficacious in sensitizing tumors to conventional chemotherapy in animal models (Fig4). We are now refining de design of therapeutic agents based on these findings in order to target simultaneously several of the factors that maintain tumor growth. These include, besides the functions of tumor cells themselves, the properties of the microenvironment around them, which consists of cellular (stromal cell, immune cells) and noncellular components. All these components are important for tumor progression and are susceptible of being used for treatment.
3. Using this knowledge for the design of innovative therapeutic strategies.
It has been known for many years that a subset of ion channels, so called voltage-gated ion channels, detect changes in the electrical potential between the inside and the outside of the cell membrane. The inside of the cells is electrically more negative than the outside, generating the so-called membrane potential in the range of -30 to -100 mV. Given the thickness of the plasma membrane, this “small” difference represents a field of 100,000 Volt/cm; to give an impression of the field intensity, a lightning during a thunderstorm generates a weaker field. Voltage-gated ion channels respond to changes in the membrane potential by allowing the flow of specific ionic species. It is relatively well understood how the protein senses the changes in potential, and significant advance has been made with respect to the mechanisms that “gate” the channel and how the ions actually cross between both sides of the membrane (Fig5). However, the link between the two events still poses relevant questions. This is important not only from an academic point of view, but also because interfering with this particular process offers the possibility to generate more potent and specific agents to treat diseases linked to the malfunction of channels. We apply biophysical and genetic techniques to study the parts of the protein that couple voltage sensing to permeation, with special emphasis on the role of intracellular domains that had been previously overlooked. It had been assumed for a long time that only those parts of the protein embedded in the plasma membrane or in its immediate proximity are relevant for electromechanical coupling, We could challenge this view using as a model Kv10.1, whose large intracellular domains not only participate in coupling, but can substitute the transmembrane segments in this function.