Neuronal Cell Biology
Publications:
http://www.ncbi.nlm.nih.gov/pubmed/?term=Kilimann+MW

Supramolecular organization of neurotransmitter and hormone release sites
Neurotransmitter release is very fast—an electrical signal can trigger membrane fusion in less than 1 ms—but at the same time highly controlled and regulated. This requires the precise interplay of hundreds of different proteins at specialized patches of the presynaptic plasma membrane known as active zones. Modulating the quantities and properties of active zone proteins fine-tunes synaptic signaling for information processing and memory tasks.

Fig. 2:
Different sequence regions of active-zone proteins reside in different locations at neurotransmitter release sites.
A-D: Immunogold electron-microscopic data;
E: schematic representation derived from these data.
Many proteins that contribute to neurotransmitter release have been identified in recent years, but our understanding of the mechanistic interplay between these proteins is still in its infancy. Moreover, it appears that these molecules are assembled in an organized fashion that enhances the speed and precision of protein interactions and turns them into small “molecular machines.” Electron microscopists discovered half a century ago that specific staining techniques can visualize presynaptic aggregates of regular size, shape, and spacing. Many researchers suspect that these aggregates, called dense projections, represent modular, structured assemblies of the neurotransmitter release machinery.
Aczonin alias Piccolo, Bassoon, CAST, Munc13, and RIM are among the presynaptic proteins that participate in neurotransmitter release. These molecules are large and complex (Aczonin and Bassoon are among the largest proteins known, with lengths of 5000 and 4000 aa, respectively), and can bind to many other proteins including each other, giving rise to a highly interconnected and interactive supramolecular assembly.
My laboratory discovered Aczonin (Wang et al., J Cell Biol 147:151-162; 1999) and identified a multi-protein complex encompassing domains of Aczonin, Bassoon, CAST and RIM which converge on the N-terminal domain of Munc13-1 (Wang et al., J. Neurosci. 29:12584-12596; 2009). I believe that this complex is one focal point for the higher-order organization of the protein constituents of neurotransmitter release sites.
By high-resolution immunogold electron microscopy, we have mapped on the nanometer scale how the large polypeptide chains of Aczonin and its interaction partners are arranged within the multiprotein array of the active zone ("in-situ topology") (Limbach et al., PNAS 108:12579-80, E392-E401; 2011).
Future molecular and electron-microscopic work aims to elucidate further how these proteins are spatially arranged at neurotransmitter and hormone release sites, and how this enables them mechanistically to function as subunits, like cogwheels, of a supramolecular machine.
The BEACH protein family: a novel molecular principle in subcellular protein targeting and human diseases
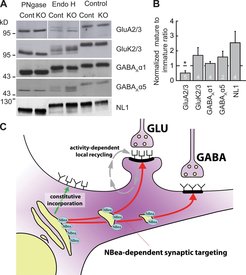
The BEACH domain is the hallmark of a protein family which expanded from a single progenitor in yeasts to 6-8 members in multicellular organisms as diverse as Dictyostelium, C. elegans, Drosophila, plants and vertebrates. Most BEACH proteins are large (~3000 amino acids [aa]) and share a domain architecture in which the highly conserved BEACH domain (~280 aa) is followed by a WD repeat at the C-terminus, whereas the N-terminal sequence regions are more divergent. Since the identification of this protein family in 1997, little has been learned about their functional mechanisms at the molecular level. Deficiency mutants of various BEACH proteins were characterized in humans, mice, flies, worms, plants, slime molds and yeast, displaying complex phenotypes of various degrees of severity. As a common denominator, a role in the dynamics of endomembranes (often including lysosomes, autophagosomes and secretory granules) and membrane proteins seems to be emerging. BEACH proteins are found in all eukaryotes, and they have expanded into families in all multicellular organisms. This indicates an important biological function for them, but it remains difficult to define this function, given the complexity of their deficiency phenotypes. I believe that they constitute a novel molecular principle in the subcellular sorting and/or targeting of many proteins, and most observations point toward the targeting of membrane proteins (intrinsic as well as lipid-anchored). Much remains to be discovered about this fascinating protein family, with many disease involvements, at the physiological, cell-biological and molecular levels.
My laboratory discovered the BEACH family member, Neurobeachin (Wang et al., J Neurosci 20:8551-8565; 2000), and continues to study Neurobeachin (NBEA) and its closest relative within the BEACH family, LRBA, by molecular, cell-biological and genetic approaches. We have generated gene knockout mice for both proteins. NBEA-KO mice die at birth and display marked perturbations of synaptic functions, both pre- and postsynaptically (Medrihan et al., J Physiol 587:5095-5106; 2009), including the mistargeting of several ionotropic neurotransmitter receptors (Nair et al., J Cell Biol 200:61-80; 2013; Gromova et al., Cell Rep 2018). LRBA-KO mice are viable but display perturbations of various sensory and gastrointestinal functions consistent with defects of subcellular protein targeting (Kurtenbach et al., Sci Rep 2017; Vogl et al., EMBO Rep 2017). Ongoing work aims to understand the molecular mechanisms through which NBEA and LRBA exert these effects on protein targeting.
Paralemmins: a new molecular principle in plasma membrane dynamics and cell shape control
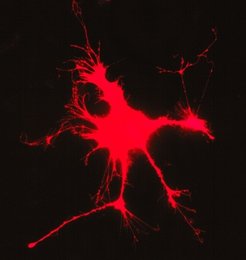
Paralemmins are a family of four homologous proteins which has been conserved throughout vertebrate evolution (Hultqvist et al., PLoS One 7:e41850; 2012), but their biological functions and molecular mechanisms have remained elusive. At the molecular level, they may have a role in the organization of membrane nanodomains by laterally clustering other membrane proteins. Results from cell-biological studies and the characterization of Paralemmin-1 KO mice indicate a role in plasma membrane dynamics (Kutzleb et al., J Cell Biol 143:795-813; 1998), in the morphogenesis of lymphatic vessels (Albrecht et al., Angiogenesis 16:795-807; 2013), and in the evolutionary expansion of the primate neocortex (Kalebic et al. Cell Stem Cell 2019).
Glycogen storage diseases
My laboratory previously identified the molecular bases of several genetic disorders of glycogen metabolism (Kilimann & Oldfors, J Inherit Metab Dis 2015). Ongoing work aims to identify the genes responsible for glycogen storage diseases of heart and skeletal muscle which are not yet understood (Krenn et al., J Neurol 2018).